Middlebury Tick Researchers Investigate the Complex Ecological Factors Driving the Rise of Lyme Disease
| by Gaen Murphree
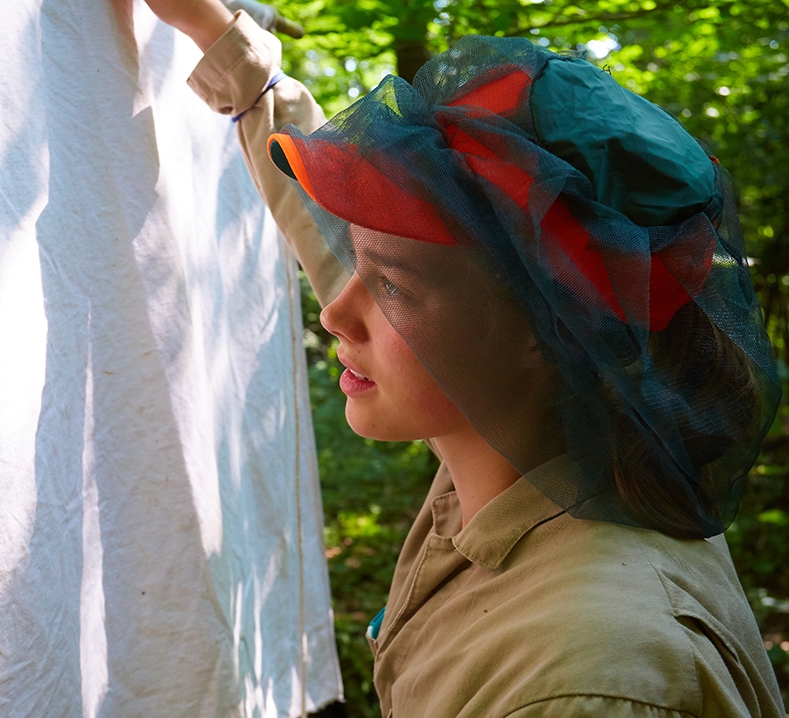
Weekday mornings find biology professor David Allen and his intrepid crew of student researchers out in the woods, trolling for ticks.
“I don’t love having to handle live ticks. I mean, I’m used to it,” says conservation biology major Robert Cassidy ’19, who admits that when spending hours scouring the woods for ticks, “the feeling is a lot like schadenfreude, where you’re excited when you find ticks … but you know it’s not good for anyone if you find a lot of ticks.”
2018 marks the third summer of Allen’s continuing study of the ecology of Lyme disease. A forest ecologist with a deep grounding in mathematics, Allen’s research involves fieldwork, lab work, and developing a mathematically driven model to help analyze, determine, and quantify the factors driving the continued rise of tick-borne illnesses.
“Tick-borne diseases are interesting because we have a very complex system where lots of different factors are interacting: climate, host community, vegetation,” said Allen. “And all these things can affect both the population of the vector, the tick, but also the persistence of the bacteria within the system. So I’m trying to look at how both abiotic drivers—like climate—and biotic drivers affect this vector-borne disease.”
Step one is counting ticks.
Each morning, Allen and his team suit up in light-colored coveralls and head out to one of Allen’s 14 designated research sites.
Once on site, the researchers split up, each armed with a one-meter square of white canvas mounted on a wooden dowel.
“It’s pretty low tech, but it’s fast, it’s cheap, and it works,” noted Allen, of the team’s drag-and-count technique.
Each site is carefully subdivided, marked by small red and yellow flags, and each subplot is further keyed to vegetation type and density. Sites range from low to high elevations (Allen uses elevation as a stand-in for climate) and are anywhere from 62 to around 42,000 acres, with large and small sites at both high and low elevations.
To catch ticks, a researcher slowly and methodically walks a subplot, dragging the canvas square along the forest floor. Every 10 meters, he or she stops, spreads out the canvas, and inspects it closely. Some kneel and peer; some spread the canvas against a tree trunk to catch the light. All find and count every single tick. Nymphs and adults get put in small plastic vials, labeled, and taken back to the lab for further research. Larvae get stuck to a piece of tape and counted.
Allen’s technique for distinguishing larvae from specks of dirt? “Blow on it and see if it crawls.” Ticks respond to movement, heat, and carbon dioxide.
Afternoons are spent back in the lab. Each tick is put under a microscope, pierced in the gut (where Borrelia burgdorferi resides) with a surgical needle, and tested for the Lyme-causing bacteria. Further tests then reveal which of the 19 possible Borrelia strains found in the Northeast an infected tick was carrying. Many strains can be correlated to specific host animals; some are more virulent to humans than others.
Out in the field, not just any old tick will do. Allen and crew are only looking for Ixodes scapularis (commonly known as the blacklegged tick, or deer tick), the tick that spreads Lyme disease in the Northeast, mid-Atlantic, and north-central states like Wisconsin and Minnesota.
“It takes a little while to learn to identify them quickly, but after that it’s pretty straightforward,” said biology major Maisie Anrod ’19.
In Vermont, the Ixodes life cycle takes two years to complete. In year one, the larvae hatch, feed, and molt into nymphs. In year two, the nymphs feed and molt into adults; the new adults then find a host and feed. Most typically, adults mate on the host, males die after mating, and females overwinter to lay eggs the next spring … and the cycle starts all over again. At each life stage, a tick feeds only once. In Vermont, larvae tend to hatch in late summer. Nymphs—which transmit the most Lyme to humans because they’re more difficult to detect—are most active early to mid-summer. Adults are most active in the fall.
Larvae are born free of Borrelia; so to be Lyme vectors ticks must acquire Borrelia from host animals. Larvae and nymphs prefer to feed on smaller animals, like mice. Adult ticks prefer larger mammals, like deer. Some animals are better Borrelia hosts and transmitters than others. Top dog in the Northeast is the white-footed mouse, Peromyscus leucopus, which is 1.5 times likelier to transmit B. burgdorferi than Eastern chipmunks, six times likelier than Eastern gray squirrels, and a whopping 35 times likelier than possums, according to some studies. (Possums, curiously enough, emerge as unlikely heroes in the battle against Lyme because in addition to low incidence for carrying the bacteria they are also great groomers and thus far likelier to bring the tick life cycle to a biting end.)
Since first being identified near Lyme, Connecticut, in 1975, Lyme has emerged as vector-borne public health enemy number one.
“The intersection of public health and conservation is really interesting,” said conservation biology major Harper Baldwin, who, along with the rest of Allen’s research crew, appreciates the real-world applicability of the Lyme ecology project. Evan Fedorov, a soon-to-be sophomore planning to major in molecular biology and biochemistry, noted that he was interested in the tick research because of his interest in infectious diseases: “Dave’s research is so relevant to Vermont and to public health, so for me it was a great fit.”
Tick-, flea-, and mosquito-borne illnesses have tripled since 2004, according to a May 2018 report from the Centers for Disease Control and Prevention. Lyme is by far the most common, accounting for 82 percent of all tick-borne illnesses and 63 percent of all vector-borne illnesses. While just 30,000 cases of Lyme are reported each year in the United States, the CDC estimates actual numbers at around 300,000.
Allen and other scientists continue to ask, “Why?”
Possible culprits include a warming climate and forest fragmentation, leading to loss of biodiversity. While mice, for example, do well in fragmented ecosystems, many others do not. Fewer foxes or owls mean a lot more mice—more ticks and more disease.
But the picture is far from complete.
Since 1975, Lyme has spread predominantly north from Connecticut to Maine (now top state for Lyme incidence, with Vermont close behind), and to Wisconsin and Minnesota. But what part of seasonal temperature variation formerly limited Ixodes’s range? Cooler summers? Warmer winters? Both? What part do rainfall, humidity, and other weather patterns play? Last summer, one of the rainiest Junes on record, Allen counted double the ticks; this year numbers have dropped to previous levels. Allen and team are building “tick hotels” this summer to see how ticks fare when exposed to different levels of humidity: desiccation can mean mortality. And what about “questing” behavior, the way ticks cling to vegetation when they crawl up out of the leaf litter, lurking for a host?
Last year a student researcher discovered that—contrary to the literature—ticks preferred conifer to deciduous leaf litter. Was that because of the record rainfall? Allen has found that ticks don’t like to quest from ferns. Why? A recently graduated senior researched Borrelia rates in two identical-looking species of mice. Taking mouse ear clips to genetically identify each species, she determined that deer mice have an even higher infection rate than white-footed mice. So did previous studies ignore the mouse doppelganger?
Understanding the ecological complexities, said Allen, will give humans better tools for predicting, controlling, and preventing the spread of Lyme disease.
“We try to tease apart those potential covariants,” said Allen, noting that “ecology is a very complicated place to do modeling. Very small systems and very large systems,” like those studied by moecular biologists, physicists, and astronomers, “are more orderly. Things in the middle don’t often follow the rules as much.”